(NSF) Contacting and Solidification in Casting-by-Design (2004-2007)
B.G. Thomas and A. Sundararajan
Paul Steen, Cornell University INC
National Science Foundation DMI 04-23794, Continuous Casting Consortium
National Science Foundation Support
DMI 04-23794
10/1/2004 to 9/30/2007
NSF Program Director: Jian Cao, (703) 292-7088
NSF Grants Official: Barbara A. Brooks, (703) 292-8213
Organizational Partners
Cornell University
Professor Paul Steen from Cornell University is the Principal Investigator for this collaborative NSF grant. His grad students, Cormac Byrne and Eric Theisin have been instrumental in providing experimental data to UI graduate student Aravind Sundararajan who has worked on the development and application of a mathematical model of the Al-7% Si strip-casting process of interest.
Continuous Casting Consortium
The Consortium, comprised of a number of steel companies has devoted resources to simulate phenomena related to initial solidification which resulted in findings crucial to the success of this project.
Project Summary
The continuous casting of thin aluminum strip with a single-wheel melt spinning process offers great potential for low-cost production of finished products with unique surface textures. To perfect this process requires fundamental understanding of the phenomena which control solidification shape, including flow oscillations in the melt pool, meniscus interaction with the wheel surface, intermittent solidification against the moving wheel, and thermal distortion. Aided by measurements by collaborators at Cornell, advanced computational models are being applied at UIUC to achieve this new understanding. Recent simulations match the contoured shape of the strip surface, based on the initial shape of the solidified meniscus, which is also the subject of model investigation.
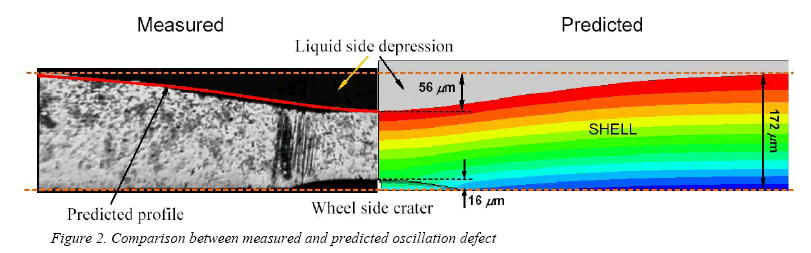
Summary of Activities Click here full Activities PDF (130 KB)
The continuous casting of thin aluminum strip with a single-wheel melt spinning process offers great potential for low-cost production of finished products with unique surface textures. To perfect this process requires fundamental understanding of the phenomena which control solidification shape, including flow oscillations in the melt pool, meniscus interaction with the wheel surface, intermittent solidification against the moving wheel, and thermal distortion. This was done through a three-step process as given below:
1) Development of a quantitative, computational model of heat transfer and solidification coupled with the transient flow of molten aluminum during the melt spinning of aluminum.
2) Validation of the model through extensive comparison with experiments performed at Cornell University under the direction of PH Steen.
3) Application of this model to improve understanding of the heat transfer during the process thereby develops a fundamental mechanism governing the relationship between various process parameters.
Melt-spinning process can be used to cast amorphous metallic glass ribbons or thin metal strips with fine microstructure and properties. The strip produced during this process has several different types of observable surface defects. These include cracks, holes, hot tears, segregation and surface depressions. If the surface depressions can be controlled, this process could be an economical way to produce strip product with textured surfaces embossed with text and images.
Different techniques can be used to produce strips with textured surfaces. These include laser interaction with the melt-pool, meniscus fluctuations from vibrations of the melt pool, and treating the wheel surface either thermally (such as via laser scanning) or physically, such as via coating deposits. For example, imprinting a layer of low-conductivity BN onto the wheel can act as an interface surface depression that transfers a ‘negative’ template from the substrate to the product during solidification. Surface depressions include longitudinal depressions along the casting direction and transverse depressions across the width of the strip. A depression on the substrate surface causes a local increase in thermal resistance at the interface between the strip and the wheel (wheel side surface) and thus slows the heat transfer and local solidification rate. This produces a corresponding deeper depression on the opposite upper surface of the strip (liquid side surface) which translates into discernible thickness variations. In order to quantify the effect of these interfacial depressions, a thorough understanding of the heat transfer phenomena occurring during this process is essential.
Findings Click here for a PDF version (143 KB)
A simple, yet accurate numerical model of the planar-flow melt-spinning process for Al-7%Si alloys on copper wheels: STRIP1D has been developed. The model include a realistic treatment of fluid flow and heat transfer in the melt pool, coupled with transient heat transfer and solidification of the strip and transient heat conduction within the wheel. Simultaneous predictions of transient strip thickness, SDAS, cooling rate, strip surface temperature and transient wheel temperature have been validated using experimental data measured at Cornell and excellent agreement has been observed. Two- and three-dimensional transient heat-transfer models of the planar-flow melt-spinning process have been developed using ABAQUS and validated with STRIP1D. The effect of process conditions including casting speed, puddle length, gap height, superheat and interfacial gaps on the heat-transfer occurring during this process have been investigated using these models. A method to quantify the surface depressions observed in melt spinning has been developed and validated using experimental measurements which reveals the mechanism of their occurrence. The following conclusions arise from this study.
- The superheat-flux method developed has been validated using multi-dimensional transient heat transfer models.
- Heat transfer across the wheel-strip interface governs solidification in the strip and heat transfer to the wheel. A new time-dependent model for interfacial heat transfer coefficient is proposed.
- In addition to controlling flow rate, and thereby strip thickness, a decrease in gap height seems also to decrease the interfacial heat transfer coefficient, perhaps due to increasing the oscillations in the puddle.
- Strip solidification depends greatly on residence time. As the contact time in zone I increases, the strip thickness increases.
- The observed non-classical strip growth profile for different solid fractions is steep with similar steep temperature contours almost parallel to each other. Also, the strip is mushy even after it enters Zone II and rapidly becomes fully solid near the end of Zone II.
- Parametric studies investigating the effects of various process variables; casting speed, gap height, puddle length, superheat and interfacial depressions on heat transfer have been determined.
- The gap height controls both the strip thickness and the heat transfer from the strip to the wheel, which together determine the puddle length.
- For all other conditions kept the same, an increase in superheat decreases the strip thickness because more heat enters the solidifying strip. If the superheat is very low, the strip might start solidifying at the nozzle resulting in freeze-up.
- Interfacial depressions on the wheel side of the strip interfere with the heat transfer to the wheel and decrease the local solidification rate resulting in an equivalent corresponding depression on the liquid side of the strip.
- The melt-spinning process is flow-rate controlled unlike the strip-casting process, which is heat-transfer controlled where the flow rate is controlled by thickness.
- Together, the STRIP1D and ABAQUS models comprise a powerful tool to study these processes. This work explains the variations in the strip thickness observed in three different time / length scales.
1. Thickness generally decreases with time during the entire cast, due mainly to decreasing gap height as the wheel expands, and also due to wheel heat-up.
2. Thickness variations with the frequency of the wheel rotation are caused by gap variations due to slightly non-circular wheel shape.
3. Small, closely-spaced transverse depressions occur due to the entrapment of air at the strip-wheel interface, owing to oscillation of the melt pool menisci. They can be predicted using a 3-D model that matches experimental measurements.
NSF Publications
Steen, P.H., B.G. Thomas, and S.P. Baker, “Collaborative Research: Contacting and Solidification in
Casting-by-Design,” Proceedings of 2006 NSF Design, Service, and Manufacturing Grantees and Research
Conference, St. Louis, Missouri, 2p, July 24-27, 2006.Click here for a PDF version (124 KB)
Sengupta, J., H.-J. Shin, B.G. Thomas, and S.-H. Kim, “Micrograph Evidence of Meniscus Solidification
and Sub-Surface Microstructure Evolution in Continuous-Cast Ultra-Low Carbon Steels,” Acta Materialia,
54:4, 1165-1173, February 2006. Click here for a PDF Version (1.08 MB)
Lee, G.-G., B.G. Thomas, H.-J. Shin, S.-K. Baek, C.-H. Choi, D.-S. Kim, S.-J. Yu, and S.-H. Kim,
“Microstructure Near Corners of Continuous-cast Steel Slabs Showing Three-dimensional Frozen
Meniscus and Hooks,” Acta Materialia, 55:20, 6705-6712, Dec. 2007 Click here for a PDF Version (1.62 MB)
J. Sengupta and B.G. Thomas, “Visualization of Hook and Oscillation Mark Formation Mechanism in
Ultra-Low Carbon Steel Slabs During Continuous Casting,” JOMe, (Journal of Metals – electronic
edition), December 2006 (http://www.tms.org/pubs/journals/JOM/0612/Sengupta/Sengupta-0612.html) Click here for a PDF Version (1.90 MB)
J. Sengupta and B.G. Thomas, “Effect of a Sudden Level Fluctuation on Hook Formation During
Continuous Casting of Ultra-Low Carbon Steel Slabs,” MCWASP XI Conference, edited by C.Z. Gandin,
M. Bellet, and J.E. Allision, Opio, France, 727-736, May 28-June 2, 2006. Click here for a PDF Version (1.29 MB)
|